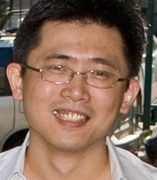
Chieh Chang, PhD
Associate Professor
Biological Sciences
Contact
Building & Room:
4152 MBRB
Address:
900 S. Ashland Ave
Office Phone:
Email:
Related Sites:
About
The research in Dr. Chang's laboratory addresses these questions: How do neurons regulate transition of sequential events in neuronal connectivity from axon pathfinding to synapse formation? How do neurons regenerate and repair themselves after injury? How do engulfing cells clean up neuronal “waste” after neuronal degeneration? How are neuronal degeneration and regeneration related? How does age influence the intrinsic nerve growth ability? What mechanisms govern dendritic arborization of the nociceptive neurons to ensure uniform sensory coverage of skin that envelops the entire animal body? Dr. Chang asks these questions mainly in the context of nematode C. elegans with an overarching goal of establishing basic principles underlying development and regeneration of neural circuits that can be applicable to other model organisms. Dr. Chang has studied signaling mechanisms that control gene expression, organogenesis, nerve pathfinding, and nerve regeneration in C. elegans for nearly nineteen years. Recently, Dr. Chang's lab identified several timing mechanisms regulating orderly neuronal connectivity and regeneration change.
Research Directions
Forty percent of genes identified in the human genome have a homolog in C. elegans. Genes that allow neurons to connect with each other to form functional neural circuits are highly conserved between C. elegans and humans. Dr. Chang’s lab studies mechanisms regulating neuronal connectivity and regeneration in C. elegans. The molecules that guide neuronal connectivity and regenerate nerves in C. elegans are similar to those that used in human. Thus, what Dr. Chang's lab learns in C. elegans will likely be relevant to the development and regeneration of the human nervous system.
Nerve Pathfinding
During development of the C. elegans nervous system, axons of many neurons, including the anterior ventral microtubule (AVM) axons, are guided to the ventral nerve cord by the UNC-6 (netrin) attractant recognized by its receptor UNC-40 (DCC). Upon reaching the ventral nerve cord, the AVM axon changes its trajectory and moves anteriorly to the nerve ring, a neuropil generally viewed as the animal’s brain. Axons are attracted to targets, but must switch their responsiveness upon arrival so that they are no longer sensitive to guidance cues and can proceed with synapse formation. In Drosophila and vertebrates, netrin signaling is inhibited by slit-induced Robo receptor binding to netrin receptor DCC. However, it is unclear how the netrin signaling is inhibited in C. elegans AVM neurons at targets. Dr. Chang's lab is using a genetic and optical approach to identify molecular mechanisms that inhibit netrin attraction.
Nerve Regeneration
The C. elegans nervous system is composed of 302 neurons with a complete map of all axon trajectories and synaptic connections. The transparency and small size of C. elegansallow us to visualize axonal development and axonal regeneration using time-lapse fluorescent microscopy as well as perform axotomy on any neurons with femtosecond laser ablation in live animals. Femtosecond laser ablation is a new optical scalpel with exquisite precision and reproducibility. The nanometer precision of femtosecond laser ablation as well as the million-fold shorter exposure interval allow us to snip individual nerve fibers without collateral damages to the cell body or neighboring fibers.
Using C. elegans as a model organism to study nerve regeneration enables Dr. Chang's lab to identify several regeneration patterns that are conserved between C. elegans and vertebrates. For example, Dr. Chang's lab observes in C. elegans the dichotomy of robust regeneration in the peripheral nervous system versus nonregenerating neurons in the central nervous system. In addition, like vertebrate neurons, C. elegans neurons lose nerve growth ability as they age, but it is not known why. Many of the positive regulators of nerve growth have been identified and well studied. Dr. Chang's major goal is to test a related hypothesis: that there are also negative regulators of nerve growth that limit the brain’s ability to grow out nerves. Are there more of these negative regulators in an aging brain than in the baby’s brain? If such molecules exist, then blocking their function in the aging brain might rejuvenate neurons to a growing state in which neurons can regenerate better. This project has the potential to open the new door for treatment of neurodegenerative diseases of aging by harnessing hidden neuronal ability to reorganize itself.
Nerve Degeneration
Dr. Chang's lab is also interested in understanding how engulfing cells help clean up neuronal “waste”. In mammals, flies, and worms, when a neuron’s connection is injured, it degenerates and sheds debris. When that happens, engulfing cells move in the injury site and remove these wastes. Dr. Chang wants to understand why it is important to remove nerve debris and to identify the mechanisms that allow engulfing cells to sense debris and engulf them. Nerve debris can also be generated during developmental pruning, which is a process widely used for the refinement of the neural circuit assembly. Dr. Chang's lab is investigating whether similar mechanisms are used for removal of these early neuronal wastes.
Selected Publications
(Complete list of publications on Google Scholar)
- Suzuki N, Zou Y, Sun HS, Eichel K, Shao M, Shih M, Shen K, Chang C. Two intrinsic timing mechanisms set start and end times for dendritic arborization of a nociceptive neuron. Proc. Natl. Acad. Sci., Dec. 2022
- Zou Y, Shih M, Chiu H, Ferreira T, Suzuki N, Zou W, Chuang C-F, Chang C. The kpc-1 (furin) 3’UTR promotes dendritic transport and local translation of mRNAs to regulate dendrite branching and self-avoidance of a nociceptive neuron. BioRxiv, 2021.08.03.453128 [Preprint]
- Shih M. and Chang C. Brain-wide identification of LIN-41 (TRIM71) protein-expressing neurons by NeuroPAL. microPublication Biology, 10.17912/micropub.biology.000472, 2021
- Chang C*, Hisamoto, N*. Engulfment genes promote neuronal regeneration in C. elegans: Two divergent but complementary views. BioEssays, 1900185, 2020. *Co-corresponding authors
- Alqadah, A., Hsieh, Y.-W., Xiong, R., Lesch, B.J., Chang C, Chuang, C.-F. A universal transportin protein diversifies olfactory neurons via specific nuclear import of a sox-2-activating factor. Proc. Natl. Acad. Sci. 116, 25137-25146, 2019.
- Chiu H, Zou Y*, Suzuki N*, Hsieh Y-W, Chuang C-F, Wu Y-C, Chang C. Engulfing cells promote neuronal regeneration and remove neuronal debris through distinct biochemical functions of CED-1. Nature Communications, 9, 4842, 2018.
- Ivakhnitskaia E, Hamada K, Chang C. Timing Mechanisms in Neuronal Pathfinding, Synaptic Reorganization, and Neuronal Regeneration. Development Growth & Differentiation, 58, 1-6, 2016.
- Dong X, Chiu H, Park YJ, Zou W, Zou Y, Özkan E, Chang C*, Shen K*. Precise regulation of the guidance receptor DMA-1 by KPC-1/Furin instructs dendritic branching decisions. eLife, 5, e11008, 2016. *Co-corresponding authors
- Alqadah A, Hsieh Y-W, Vidal B, Chang C, Hobert O, Chuang C-F. Postmitotic diversification of olfactory neuron types is mediated by differential activities of the HMG-box transcription factor SOX-2. EMBO Journal, 34, 2574-2589, 2015.
- Chiu H, Alqadah A, Chang C. The role of microRNAs in regulating neuronal connectivity. Frontiers in Cellular Neuroscience 7, 1-6, 2014.
- Chiu H, Chang C. Rejuvenating nerve cells in adults. Aging 5, 1-2, 2013.
- Zou Y†, Chiu H†, Zinovyeva A, Ambros V, Chuang C-F, Chang C. Developmental decline in neuronal regeneration by the progressive change of two intrinsic timers. Science 340, 372-376, 2013. †Equal contribution.
- Recommended by Faculty of 1000; Featured in “Perspective: Heterochronic genes turn back the clock in old neurons” Nix P, Bastiani M, Science, 340, 282-283, 2013; Highlighted in “Editors’ Choice: Turn Off Youth” Hines P. J., Science Signaling, 2013.
- Zou Y, Chiu H, Domenger D, Chuang C-F, Chang C. The lin-4 microRNA targets the LIN-14 transcription factor to inhibit netrin-mediated axon attraction. Science Signaling 5, research article ra43, 2012.
- Chiu H, Alqadah A, Chuang C-F, Chang C .C. elegans as a genetic model to identify novel cellular and molecular mechanisms underlying nervous system regeneration.Cell Adhesion & Migration 5, 387-394, 2011.
- Gabel CV, Antoine F, Chuang CF, Samuel ADT, Chang C. Distinct cellular and molecular mechanisms mediate initial axon development and adult-stage axon regeneration in C. elegans. Development 135, 1129-36, 2008.
- Chang C, Adler C, Krause M, Clark S, Hao J, Gertler F, Tessier-Lavigne M*, Bargmann CI*. MIG-10/Lamellipodin and the lipid modulator AGE-1/PI3K promote axon guidance and outgrowth in response to Slit and Netrin. Current Biology 16, 854-62, 2006. *Co-corresponding authors
- Chang C, Yu TW, Bargmann CI*, Tessier-Lavigne M*. Inhibition of Netrin-mediated axon attraction by a receptor protein tyrosine phosphatase. Science 305, 103-6, 2004. *Co-corresponding authors Recommended by Faculty of 1000
- Chang C, Werb Z. The many faces of metalloproteases: cell growth, invasion, angiogenesis, and metastasis. Trends in Cell Biology 11, S37-43, 2001.
- Chang C, Hopper NA, Sternberg PW. Caenorhabditis elegans SOS-1 is necessary for multiple RAS-mediated developmental signals. EMBO Journal 19, 3283-94, 2000.
- Chang C, Newman AP, Sternberg PW. Reciprocal EGF signaling back to the uterus from the induced C. elegans vulva coordinates morphogenesis of epithelia. Current Biology 9, 237-46, 1999.
Education
Postdoctoral Fellow, Stanford University, UCSF, and Rockefeller University
PhD, California Institute of Technology, Pasadena, CA